What is the Fatigue Testing?
- Video about Fatigue Testing
- Representation of Fatigue Testing
- Metallic Materials
- Polymer Materials
- Ceramic Materials
- Composite Materials
- ISO Standards for Fatigue Testing
- ASTM Standards for Fatigue Testing
What is the Fatigue Testing?
Fatigue Testing is a method used to evaluate how a material behaves under repeated stress and cyclic loading. It helps in determining the material’s endurance limit and fatigue life, identifying how long it can withstand fluctuating stresses without failure. This testing is crucial in various industries to ensure material reliability, safety, and performance over time.
Video about Fatigue Testing
Representation of Fatigue Testing
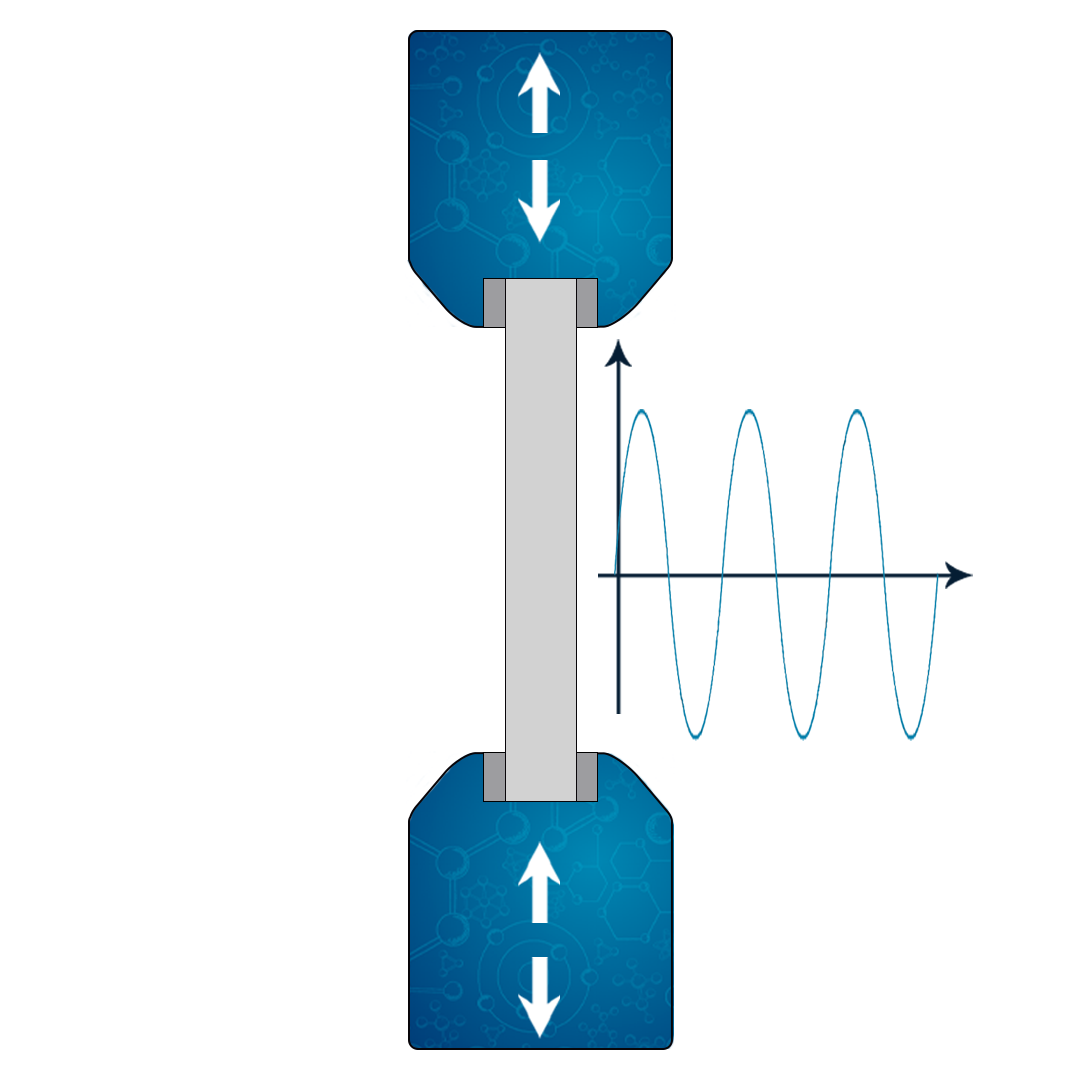
Fatigue Testing measures several critical properties of a material under cyclic loading conditions, including:
- Endurance Limit: The maximum stress a material can withstand without failure for an infinite number of cycles.
- Fatigue Life: The number of cycles a material can endure before failure occurs.
- Stress vs. Strain Relationship: Understanding how stress and strain are related during the cyclic loading provides insights into material elasticity and plasticity.
- Crack Initiation and Propagation: Determining where cracks begin and how they spread during cyclic loading, offering insights into potential failure points.
- Material’s Damping Characteristics: Analyzing how a material dissipates energy during cyclic loading, which can impact its long-term performance.
By measuring these aspects, Fatigue Testing provides vital information for material selection, design considerations, safety protocols, and overall performance evaluation in various applications and industries.
What is the purpose of Fatigue testing?
The purpose of Fatigue Testing is multifaceted, playing a crucial role in various aspects of material science and engineering. Here’s a detailed look at its main objectives:
- Understanding Material Behavior: Fatigue Testing helps in understanding how a material behaves under cyclic or repeated loading, revealing its characteristics such as endurance limit, fatigue life, and the nature of crack initiation and propagation.
- Predicting Material Failure: By simulating the repeated stresses a material will face in real-world applications, Fatigue Testing allows engineers and scientists to predict when and how a material might fail. This understanding is vital in designing components that will not fail unexpectedly.
- Improving Material Design: Insights gained from Fatigue Testing enable engineers to optimize materials and designs, leading to products with improved performance, durability, and reliability.
- Ensuring Compliance with Standards: Different industries have specific standards that materials must meet to ensure safety and performance. Fatigue Testing ensures that materials comply with these standards, whether they are international (such as ISO) or specific to a region or industry (such as ASTM).
- Quality Control: In a manufacturing environment, Fatigue Testing serves as a quality control tool, ensuring that the materials produced meet the required specifications and performance criteria.
- Reducing Costs: By identifying potential failure points and weaknesses in materials, Fatigue Testing can lead to the selection of more suitable materials and design improvements, thereby reducing the costs associated with material failure, maintenance, and replacement.
- Enhancing Safety: In safety-critical applications such as aerospace, automotive, or construction, understanding how materials fatigue is essential to preventing catastrophic failures that could lead to accidents or loss of life.
- Supporting Research and Development: Fatigue Testing is a vital tool in research and development, enabling the creation of new materials with desired properties and the refinement of existing materials for new applications.
What are the types of Fatigue Testing?
Fatigue testing is an essential aspect of materials science, assessing how a material responds to cyclic loading. Various types of fatigue testing have been developed to mimic different loading conditions and environments that materials might encounter in real-world applications. Here’s an overview of the primary types of fatigue testing:
High-Cycle Fatigue (HCF) Testing:
- Description: Performed at low stresses and high numbers of cycles (usually more than 10^6 cycles).
- Applications: Used for materials subjected to many cycles during their lifetime, such as engine components or wind turbine blades.
Low-Cycle Fatigue (LCF) Testing:
- Description: Conducted at high stresses and low numbers of cycles (usually fewer than 10^3 to 10^4 cycles).
- Applications: Relevant for materials exposed to heavy loads, such as earthquake-resistant structures or power plant components.
Thermal-Mechanical Fatigue (TMF) Testing:
- Description: Involves simultaneous changes in mechanical load and temperature, replicating the conditions faced in applications like turbine engines.
- Applications: Crucial for materials exposed to fluctuating temperatures, like those in aerospace or automotive exhaust systems.
Multiaxial Fatigue Testing:
- Description: Subjects the material to complex loading in more than one direction, reflecting the multidirectional stresses experienced in real-life applications.
- Applications: Particularly relevant for automotive and aerospace components, where parts are subjected to diverse stress directions.
Strain-Controlled Fatigue Testing:
- Description: The strain is controlled instead of the stress, and the material is cycled through specific strains, reflecting operational conditions.
- Applications: Useful for analyzing the behavior of materials under controlled deformation, like in certain biomedical applications.
Corrosion Fatigue Testing:
- Description: Combines cyclic loading with a corrosive environment to study how corrosion affects fatigue life.
- Applications: Vital for materials used in aggressive environments like marine structures, chemical processing equipment, etc.
Fretting Fatigue Testing:
- Description: Focuses on the wear and fatigue damage that occurs at the contact area between two materials under load and slight relative movement.
- Applications: Important in understanding joint failures, such as bolted joints in automotive or aerospace structures.
Vibration Fatigue Testing:
- Description: Subjects the material to oscillatory vibrations to mimic real-world vibrational stresses.
- Applications: Relevant for components exposed to constant vibrations, such as in machinery or transportation equipment.
How to calculate the Fatigue testing?
Calculating fatigue in material testing involves a complex process, requiring knowledge of the material’s properties, loading conditions, and environmental factors. Here’s a general guide to understanding how fatigue testing may be calculated:
Define the Loading Conditions
- Stress Range (Δσ): Determine the fluctuating stress applied to the material.
- Mean Stress (σ_m): Calculate the average stress during the cyclic loading.
- Amplitude (σ_a): Calculate the stress amplitude, usually as half the stress range.
- Number of Cycles (N): Define the number of cycles the material will be subjected to.
Determine Material Properties
- Obtain the material’s S-N curve, which relates stress (S) to the number of cycles to failure (N). This curve can be obtained through experimentation or from literature for many materials.
Apply Fatigue Theories and Models
- Select an appropriate fatigue theory or model based on loading conditions and material behavior. Common models include Goodman, Gerber, or Soderberg models.
- Use these models to relate stress, mean stress, and amplitude with fatigue life.
Use Statistical Methods
- Fatigue behavior can vary between samples due to material inconsistencies, manufacturing variations, etc. Statistical methods may be applied to account for these variations.
Consider Environmental Factors
- If applicable, adjust for environmental factors like temperature or corrosion that may affect fatigue life.
Perform Analysis with Software
- Many modern engineering software tools can facilitate fatigue analysis by integrating the above factors, providing a more efficient and accurate calculation.
Validate with Experimental Testing
- Often, theoretical calculations are validated with physical fatigue testing to ensure they accurately represent real-world behavior.
Calculating fatigue testing involves a combination of material science, mechanical engineering, and often statistical analysis. Due to the complexity of real-world materials and loading conditions, it may require specialized software and collaboration between experts in various fields. Understanding these calculations is essential for design, material selection, and predicting the lifespan of components in a wide array of applications.
How to calculate the Fatigue testing?
Calculating fatigue in material testing involves a complex process, requiring knowledge of the material’s properties, loading conditions, and environmental factors. Here’s a general guide to understanding how fatigue testing may be calculated:
Define the Loading Conditions
- Stress Range (Δσ): Determine the fluctuating stress applied to the material.
- Mean Stress (σ_m): Calculate the average stress during the cyclic loading.
- Amplitude (σ_a): Calculate the stress amplitude, usually as half the stress range.
- Number of Cycles (N): Define the number of cycles the material will be subjected to.
Determine Material Properties
- Obtain the material’s S-N curve, which relates stress (S) to the number of cycles to failure (N). This curve can be obtained through experimentation or from literature for many materials.
Apply Fatigue Theories and Models
- Select an appropriate fatigue theory or model based on loading conditions and material behavior. Common models include Goodman, Gerber, or Soderberg models.
- Use these models to relate stress, mean stress, and amplitude with fatigue life.
Use Statistical Methods
- Fatigue behavior can vary between samples due to material inconsistencies, manufacturing variations, etc. Statistical methods may be applied to account for these variations.
Consider Environmental Factors
- If applicable, adjust for environmental factors like temperature or corrosion that may affect fatigue life.
Perform Analysis with Software
- Many modern engineering software tools can facilitate fatigue analysis by integrating the above factors, providing a more efficient and accurate calculation.
Validate with Experimental Testing
- Often, theoretical calculations are validated with physical fatigue testing to ensure they accurately represent real-world behavior.
Calculating fatigue testing involves a combination of material science, mechanical engineering, and often statistical analysis. Due to the complexity of real-world materials and loading conditions, it may require specialized software and collaboration between experts in various fields. Understanding these calculations is essential for design, material selection, and predicting the lifespan of components in a wide array of applications.
Fatigue testing in differents typs of materials
Fatigue testing is essential across various materials including metallic, polymer, ceramic, and composite materials. Here’s a detailed look at examples and characteristics of these materials in fatigue testing:
Metallic Materials
Metals are often subjected to fatigue testing, especially in automotive, aerospace, and structural applications.
Steel:
- Description: A widely-used alloy of iron and carbon, known for its high tensile strength and ductility.
- Fatigue Strength: Around 250-500 MPa for high-strength steels.
- Example Use: Aircraft structures, automotive components.
- S-N Curve Characteristics: May exhibit an endurance limit below which infinite life is expected.
Aluminum:
- Description: Lightweight, corrosion-resistant metal.
- Fatigue Strength: Around 50-150 MPa for common alloys.
- Example Use: Automotive body panels, aircraft wings.
- S-N Curve Characteristics: Often lacks a distinct endurance limit.
Polymer Materials
Polymers are tested for fatigue to assess their suitability in applications like medical devices, packaging, and automotive parts.
Polyethylene (PE):
- Description: Widely used thermoplastic, known for its flexibility.
- Fatigue Strength: Can vary greatly depending on type, around 5-20 MPa.
- Example Use: Plastic containers, joint replacements.
- S-N Curve Characteristics: May exhibit a continual decrease without a clear endurance limit.
Polycarbonate (PC):
- Description: Strong and transparent thermoplastic.
- Fatigue Strength: Typically around 20-40 MPa.
- Example Use: Eyewear lenses, automotive headlights.
- S-N Curve Characteristics: Similar to PE, often lacks a clear endurance limit.
Ceramic Materials
Ceramics are tested for fatigue in applications requiring hardness, wear resistance, and thermal stability.
Alumina (Al₂O₃):
- Description: A ceramic known for hardness and resistance to wear and corrosion.
- Fatigue Strength: Around 300-400 MPa.
- Example Use: Cutting tools, biomedical implants.
- S-N Curve Characteristics: Often brittle, with limited ductility.
Zirconia (ZrO₂):
- Description: Often used in its stabilized form, has excellent toughness for a ceramic.
- Fatigue Strength: Around 800-1200 MPa.
- Example Use: Dental crowns, cutting tools.
- S-N Curve Characteristics: May exhibit toughening mechanisms.
Composite Materials
Composites combine different materials to achieve desired properties and are tested for fatigue in aerospace, automotive, and sports equipment.
Carbon Fiber Reinforced Polymer (CFRP):
- Description: Combines carbon fibers with a polymer matrix, known for its high strength-to-weight ratio.
- Fatigue Strength: Can vary greatly, around 150-200 MPa.
- Example Use: Aircraft components, racing bicycles.
- S-N Curve Characteristics: Depends on fiber orientation, matrix material, etc.
Glass Fiber Reinforced Polymer (GFRP):
- Description: Incorporates glass fibers into a polymer matrix, offering good strength and flexibility.
- Fatigue Strength: Typically around 70-100 MPa.
- Example Use: Wind turbine blades, boats.
- S-N Curve Characteristics: Similar to CFRP, depends on composition.
Fatigue testing across these various material types helps engineers understand how materials will perform under cyclic loads, providing insights essential for material selection, design optimization, and failure prevention. Each material group offers unique challenges and opportunities in fatigue testing, reflecting their distinct mechanical behavior, usage, and underlying microstructures.
Parts and Components of a Fatigue Testing Machine
A Fatigue Testing Machine is a sophisticated piece of equipment designed to apply cyclic loads to a material specimen to study its fatigue behavior. Various types of fatigue testing machines are available, depending on the specific requirements of the test, such as load range, frequency, and test environment. Below are the essential parts and components found in a typical Fatigue Testing Machine:
Loading Frame
- Actuator: Applies the cyclic load to the specimen. It can be hydraulic or electromagnetic, depending on the load requirements.
- Load Cell: Measures the force applied to the specimen, ensuring that the correct load is applied.
- Crosshead: Adjusts the alignment and height of the setup to accommodate different specimen sizes.
Grips and Fixtures
- Grips: Holds the specimen securely in place during testing.
- Alignment Fixtures: Ensures that the load is applied correctly and evenly across the specimen.
Environmental Chamber (Optional)
- Chamber: Allows testing under specific environmental conditions like temperature, humidity, or corrosive environments.
- Temperature Controllers: Regulates the temperature within the chamber.
- Humidity Controllers: If needed, controls the humidity level inside the chamber.
Control System
- Control Console: Where the operator can set test parameters, such as load levels, load frequencies, and number of cycles.
- Software Interface: Modern systems may include software to design complex test cycles, monitor the test, and analyze data.
Data Acquisition and Monitoring
- Sensors: Various sensors to monitor key variables like displacement, strain, temperature, etc.
- Data Acquisition System: Records data from the sensors for later analysis.
Safety Features
- Emergency Stop Buttons: Allows quick termination of the test in an emergency.
- Protective Shielding: Protects the operator from debris in case of specimen failure.
Specimen Geometry Adapters
- Specimen Holders: Different holders and adapters may be available to accommodate various specimen shapes and sizes.
Vibration Isolation System (If Required)
- Isolation Pads or Table: Minimizes transmission of vibrations to or from the surrounding environment.
A Fatigue Testing Machine consists of various intricate parts and components working cohesively to apply controlled cyclic loads to a specimen. Understanding these parts enables more efficient use of the equipment and ensures that tests are conducted accurately and safely. The components and features may vary depending on the specific type of fatigue test being conducted and the requirements of the material and application being studied. Manufacturers and testing standards provide detailed guidelines on the correct use and maintenance of these components.
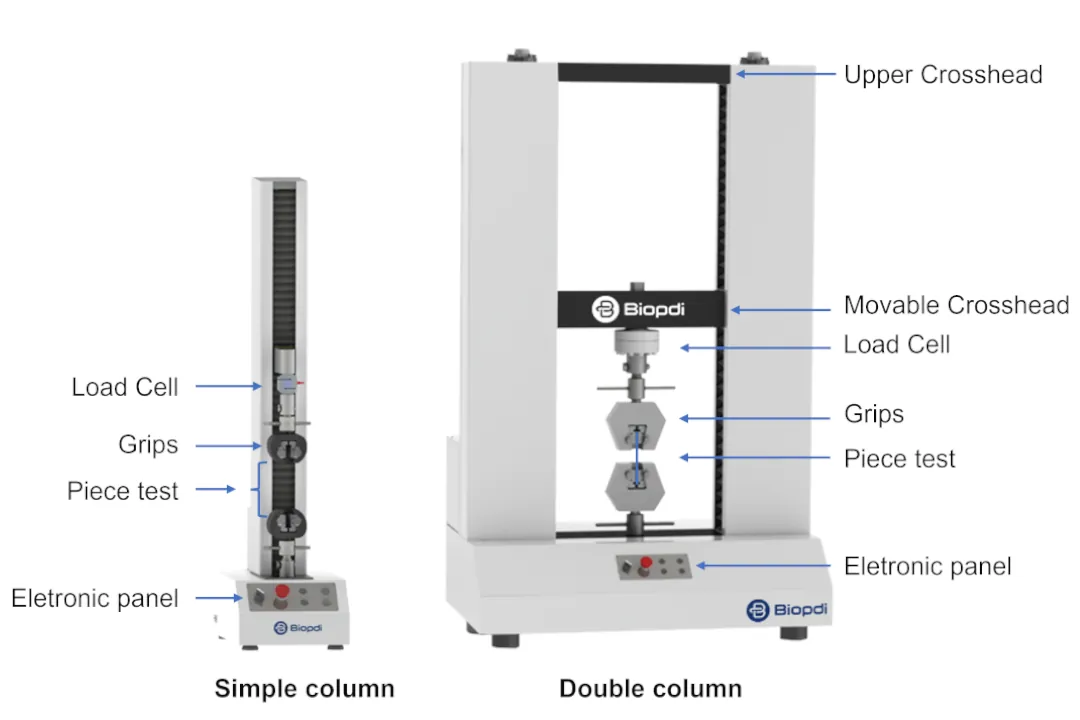
Data obtained from a Fatigue Testing
Fatigue testing using a Fatigue Testing Machine yields a wealth of data that provides valuable insights into the material’s behavior under cyclic loading. Here’s an overview of the typical data obtained:
Stress and Strain Data
- Applied Stress: The stress applied to the specimen during each cycle, including maximum, minimum, and mean stress values.
- Strain Response: The corresponding strain in the specimen, which provides information on the material’s deformation under the applied load.
- Stress-Strain Hysteresis Loops: If recorded, these loops show the material’s cyclic hardening or softening behavior.
Cycle Data
- Number of Cycles: Total number of load cycles applied to the specimen, both to failure and at various intervals during the test.
- Load Frequency: The rate at which the load cycles are applied, often measured in Hertz (Hz).
Fatigue Life and Endurance Limit
- Fatigue Life (Nf): The number of cycles to failure at a specific stress level, used to construct S-N curves.
- Endurance Limit (if applicable): The stress level below which the material can endure an infinite number of cycles without failure.
Failure Analysis
- Failure Mode: Details of how the specimen failed, such as crack initiation, crack propagation, and final fracture.
- Failure Location: The specific location of failure within the specimen, which can provide insights into stress concentrations and material defects.
Material and Specimen Information
- Material Properties: Specific details about the material, such as composition, grain size, heat treatment, etc.
- Specimen Geometry: Dimensions, shape, and other geometric details of the specimen, affecting the stress and strain distribution.
Additional Observations
- Visual and Microscopic Observations: Images, microscope photographs, or other visual data showing surface conditions, crack initiation, etc.
- Time-Dependent Behavior: If applicable, data on creep or relaxation effects within the material during the test.
The data obtained from fatigue testing is rich and multifaceted, providing a comprehensive understanding of how a material responds to cyclic loading. It informs critical engineering decisions, such as material selection, design modifications, and predictive maintenance. Properly interpreting this data requires expertise in material science and engineering, as well as adherence to testing standards and protocols. Often, specialized software is used to process and analyze the data, leading to meaningful insights into the material’s fatigue behavior.
The main standards for obtaining the Axial Torsion Test
Fatigue testing is governed by various international standards to ensure consistency, reliability, and accuracy. These standards cover different aspects of testing, including specimen preparation, test methods, and data analysis.
ISO Standards for Fatigue Testing
- ISO 1099: This standard relates to axial load fatigue testing of metallic materials and covers test methods and terminology.
- ISO 12107: Specifies statistical methods for analyzing fatigue data, including S-N curves and confidence limits.
- ISO 12110-1: Pertains to the fatigue testing of unnotched and notched specimens at ambient temperature.
- ISO 11405: Focuses on test methods for fatigue testing of dental materials, such as ceramics and polymers.
- ISO 13003: This standard is used for fatigue testing of fiber-reinforced plastics, covering aspects such as test specimen preparation and cyclic loading conditions.
ASTM Standards for Fatigue Testing
- ASTM E466: Standard Practice for Conducting Force Controlled Constant Amplitude Axial Fatigue Tests of Metallic Materials.
- ASTM E647: Standard Test Method for Measurement of Fatigue Crack Growth Rates. This standard is often applied to understand the propagation of small cracks in metals.
- ASTM E1820: Covers the determination of fracture toughness, fatigue crack growth rate, and other fracture parameters for metallic materials.
- ASTM D7791: Standard Test Method for Uniaxial Fatigue Properties of Plastics. This standard is used to evaluate the fatigue behavior of rigid and semi-rigid plastics.
- ASTM D3479: Standard Test Method for Tension-Tension Fatigue of Polymer Matrix Composite Materials. This standard provides guidelines for fatigue testing of composite materials subjected to a tensile loading condition.
Conclusion
In this text about of fatigue testing, we have understand how materials respond to repetitive stress. From understanding the fundamental purpose and types of fatigue testing, including Mechanical Fatigue, Creep Fatigue, Thermo-mechanical Fatigue, Corrosion Fatigue, and Fretting Fatigue, we’ve examined the intricate details of various materials tested and the specialized methods used.
The roles of international standards, the components and procedures of a Fatigue Testing Machine, and the essential data collected were highlighted. Additionally, we have delved into aspects like the S-N curve, surface treatments, and computer simulations that enhance the understanding of fatigue behavior.
Fatigue testing remains an indispensable tool in material science and engineering, providing vital insights that inform design, manufacturing, and maintenance practices across numerous industries.
FAQs
What tests are done to measure fatigue?
Fatigue tests include uniaxial and multiaxial fatigue testing, variable amplitude loading, resonance fatigue testing, thermal fatigue testing, and others, often adhering to specific standards such as ISO or ASTM.
Why is the fatigue test important?
Fatigue testing is crucial for understanding how materials respond to repeated loads, enabling safe and efficient design, manufacturing, and maintenance across various industries.
What are the three stages of fatigue test?
The three stages are typically: 1 – Initiation Stage: Crack forms at a defect or stress concentration; 2 – Propagation Stage: Crack grows incrementally with each load cycle; 3 – Final Failure Stage: Sudden failure occurs when the crack reaches a critical size.
What is the fatigue test for concrete?
Concrete fatigue tests evaluate how repeated loads affect concrete structures, vital for applications like bridges and roads. ASTM C666 is commonly used for such testing.
What does fatigue strength tell us?
Fatigue strength indicates the maximum stress a material can withstand for a specified number of cycles without failure, providing insights into its durability.
What is the ASTM standard for fatigue?
Several ASTM standards relate to fatigue, such as ASTM E466 for metallic materials and ASTM D3479 for composites.
What is the difference between creep test and fatigue test?
Creep testing measures how a material deforms under constant load over time, while fatigue testing examines how it responds to repeated cyclic loading.
What is the first step or procedure in fatigue analysis?
Identifying and understanding the loading conditions and material properties is typically the first step, guiding the selection of appropriate test methods and specimen preparation.
Why is fatigue difficult to measure?
Fatigue is complex due to variables like loading conditions, material properties, environmental factors, and microscopic defects, making control and measurement challenging.
What is the conclusion of the fatigue test?
The conclusion provides insights into the material’s fatigue behavior, crack initiation, propagation, failure modes, and informs decisions in design, selection, and maintenance.
What does fatigue failure look like?
Fatigue failure often shows a “beach mark” pattern with concentric ridges originating from the crack initiation point.
What causes fatigue failure?
Fatigue failure results from repeated cyclic stresses, which can lead to crack initiation and growth, culminating in sudden failure.
What is an example of a fatigue failure?
Fatigue failure in an aircraft wing due to repeated loading and unloading is an example, leading to cracks and potential catastrophic failure.